Decarbonizing Industrial Heat Means Electrifying It — Transcript Of 2nd Half Of Podcast With Paul Martin
Recently I sat down virtually with professional chemical process engineer Paul Martin of Spitfire Research on my podcast Redefining Energy – Tech to discuss how much industrial heat can be replaced with electrical heat technologies (tl’dr: virtually all of it directly). In the theme of providing transcripts of presentations I’m giving in various places for people who prefer the written word, this is the lightly edited transcript of the second half of our conversation. If you missed the first half, read or listen to it here.
Michael Barnard (MB): Hi, welcome back to Redefining Energy – Tech. I’m your host, Michael Barnard. Returning for the second half of my conversation on industrial heat with PM, chemical process engineer and chief guy at Spitfire Research.
Paul Martin (PM): There is actually a solution to this problem that doesn’t involve electricity. So if we have very inexpensive electricity coming to us part of the day, and we need heat all day, every day, or if we need heat in big batches at high powers for short periods of time, but we have electricity coming to us over longer periods of time, either of those two situations can be solved with an electrical battery, but they can also be solved with a heat battery. And there are several firms that are working on this. The one that I’m most familiar with is Antora, because they’re a customer of mine, and what they’re doing is they will take electricity whenever it’s available, and they use it to heat up a pile of graphite blocks inside an inert atmosphere.
And they recover the heat from these bricks not by basically circulating a fluid around the bricks and taking it away by conduction, but they actually have the light, the infrared light from and the visible light from these bricks shine onto collectors that then can collect that energy either as heat or as a smaller amount of electricity with the balance as heat. Now, the latter thing is kind of, well, you know, efficiency of generating electricity from heat, again, is not all that great, but, you know, if you can do it and you can also make heat at the same time, why not?
But the real benefit there is that you can either provide industry that needs heat on a steady basis with steady heat based on intermittent, inexpensive electricity, or you can draw huge amounts of energy from this pile of very hot objects to supply very large amounts of power, heating power to a process that needs intense amounts of heat for short periods of time, and then charge them up more slowly from your electrical supply. So it’s a really cool idea. And the cost per unit of delivered energy stored, ultimately, if it’s done right, should be a lot cheaper if you store it as heat than if you store it as electrical energy in a battery, at least in the short term. Over the longer term, as a battery optimist, I think that sodium-ion batteries are going to be super cheap.
And honestly, most of the other things that we use to store electrical energy, although they’re fun and interesting to work on, most of them are deader than the doornail.
MB: So let’s pull this apart a little bit, because how big is the pipe? Well, I had recent cause to look at the distribution network capacity factors for North America versus India recently, because I’m a nerd and I needed to know something for some reason or other. And I went and found that.
PM: No need to apologize to another nerd.
MB: This is my entire life. People ask me why I know all this stuff, and it’s because, well, I’m a nerd. I just need to know. I need to know everything about climate change and its solutions. I can’t help myself. But the distribution grid in the developed world is running around 50% to 60% capacity. What that means is that over a full year it could deliver 100 units of energy, but it only delivers 50 or 60. That seems like a waste, except that we get back to exactly what we’re talking about. We need a lot of power at peak period times and a lot of energy at peak period of times. And we don’t need a lot in the middle of the night, for example. And increasingly we don’t need it in the middle of the day because we’ve got the solar duck curves.
And so the distribution grid is kind of like sitting there with these massive troughs and massive peaks in the course of any given day. And now as we get to these thermal storage for industrial and domestic comfort heat, because we can actually do that with hot water heaters or thermoclines. Now if we pump heat into a big tank and then draw that heat down as needed — Harvest Thermal does that — we can actually flatten the daily capacity factor and lift it. I’m projecting we’re going to end up with about an 80% to 90% capacity factor. And the daily capacity of the distribution grid for a region for electricity will be pretty flat. It’ll just be, we’ll have EV’s charging at night. We’ll have thermal batteries charging at night. We’ll have grid storage batteries charging at night. Ditto during the duck curve and drawing down during the peak curve. So it’s going to get a really flat curve.
PM: Yeah. I think when we run into infrastructure pinches, like if one thinks of it, you know, the kind of the size of the wire that we use to carry electricity from one place to another. One of the things that you’ve talked about that I think is really pertinent and important is that we can change the type of wire that we use and by so doing increase the size of the effective size of the pipe, if you will, to carry electrons from place to place. But you’re absolutely right. We, right now, our demand and our supply don’t match very well, and we don’t pair them up with storage very much. What we do is we kind of have supply that we ramp up and down to match unpredictable demand.
You know, when everybody gets up from the Super Bowl and goes to the bathroom at the same time, and all the pumps run to refill all the toilets, you know, we tend to enforce the notion in people’s minds that electricity is just this thing that you get anytime that you plug something in or you flip a switch, as opposed to something that you have to think about. Where I see some of this flattening happening is not through storage as much as being smarter about demand. And the example I use is right now, if I were to put a load of laundry in the dryer, I have an electric dryer, as opposed to going out and hanging it on the line because I’m lazy. If I push the button on the dryer it will just start.
The dryer doesn’t know what electricity costs, and it doesn’t care. I’m supposed to know and I’m supposed to push the button at the right time. But in the future, when I push the button on the dryer will say, I’m going to start drying this load of clothes when electricity gets cheap, unless you push this button again and tell me that you need your clothes dried right now, then I’ll turn on and I’ll use expensive electricity if you’re really insistent. So a lot of this future demand, supply matching will happen as a result of smart demand and just being smarter about demand. Some of it will happen through storage and so on. But we’re well off our topic of heating.
I think as far as storage is concerned, one of the things that kind of is thrown up as not really a nirvana fallacy argument against electric heating, but as a real problem that we have to contend with in cold climates, is what the heck do we do with a massive amount of heat power? So the massive amount of heat energy per unit time that we have to deliver on the very coldest night of the year. And that is a hard problem, and it’s one that we can solve a bunch of dumb ways that seem quite impractical and that we could solve in a bunch of smart ways if were, you know, thinking about it more carefully. But frankly, in cold climates, and especially in the coldest parts of cold climates, so, for instance, northern Alberta in Canada, honestly, the most practical solution is during those coldest months, we’re probably going to still have to burn fuels. We’re probably going to burn fuels that we don’t make from electricity. We’re not likely to be burning hydrogen or things made from hydrogen. We’re likely to just keep burning fossils for the foreseeable future during those periods. But it will make a lot more sense to manage those periods and make those periods very short. And then to have electricity do the heavy lifting, the vast majority of the joules.
MB: But that’s comfort heating again. So let’s get back to industrial heating. Jan Rosenow is my next guest. And so next month is comfort heating. Let’s get back to those higher temperatures. What do we mean when we say hot? What are the gradations of hot?
PM: Well, everything from 200 to about 1000 degrees C as far as electric heating is concerned, it’s more or less the same. And basically what that means is that what we’re going to do is take a joule of electricity and turn it into a joule of heat in a resistor. And finding resistors that’ll do 1000 degrees C is not difficult. Now, you know, finding ones that will do 800 degrees C is a little easier than finding the ones that do the 800 to 1000. But even still, there are materials, metallic materials, alloys that work really well at 1000 degrees C, will heat things up to 1000 degrees C with proper design without too much difficulty. Now, sometimes heating things to those temperatures, regardless how you do it, is hard.
And there are a few occasions where we can’t just apply heat, we actually need fire. So I’ll give you an example of, one of the most obvious examples of that is cement clinkering. So when one makes cement, there are two processes.
MB: Just briefly needs the characteristics of fire, correct?
PM: Yeah. So we, there are, well, there are applications where you actually need a hot gas, you need a hot flue gas, and those are most easily supplied with fire, although you could do it another way. But when we’re talking about cement clinkering, as I mentioned, there’s two steps in cement-making. Basically, the first one is cooking off the CO2 from limestone to make lime, and that’s called calcining. And then the next step is heating up the mixture of minerals in order to form the silicate materials that are necessary for cement. And that requires very high temperatures. And the way we make those very high temperatures is actually by having a flame inside a tube that’s lined with bricks. And the bricks keep the metal tube that supports everything from getting hot.
And the heat is transferred by radiation from the flame to not just the solid material that’s rolling around inside the kiln, but to the bricks, which then transfer heat to the solid material that’s rolling around inside the kiln. And so doing that electrically is possible, but it’s challenging because you basically have to make what amounts to a flame. And there are ways to do it, but it’s rough. It’s kind of difficult. But most heating, again, isn’t cement clinkering. Most heating, interestingly, resistance heaters. It’s not.
MB: I just spent a bunch of time a couple of weeks ago talking to a person whose firm does electric plasmas. It has the characteristics necessary for that. Now when we talk about technology readiness level, it’s being done. There are cement clinker ovens that use electric plasmas, electric flames, but they aren’t just bog standard like heat pumps. They aren’t bog standard like the stuff we’re talking about under 200 degrees C. It’s not like you go to Alibaba and get a big scaled one and get a hundred delivered for North America, you know, with an order. We’re not there.
PM: There are a lot of people out there who think that the way that we do something right now is the only way that it’s possible to do it. And they’re not really looking hard at they’re not really looking very hard at the overall project and what the best solution would be under a decarbonized context. And they’re not thinking about it properly and as a consequence of drawing conclusions that are just wrong. So where I was going with this, though, is that, you know, from 200 to 1000 degrees C, it’s pretty straightforward. It’s the correct deployment of the correct design of resistance heater that’s largely going to be the solution that’s used above 1000 degrees. You’re into kind of specialty stuff. Plasmas and arcs are related, but not necessarily the same. And they’re a method that can achieve very high temperatures. Sometimes you don’t need temperatures quite so high. If you have materials that are conductive, you can use inductive heating sometimes.
MB: Let’s just talk about the most extreme. What’s the temperature of an electric plasma? What’s the temperature range for electric arcs?
PM: Oh, my goodness. 10,000 degrees C. 15,000 degrees C.
MB: Super extreme temperature of the surface of the sun.
PM: Yes, exactly. I mean, literally, when you’re talking about a plasma, you’re talking about a soup of nuclei and electrons floating around, not connected to one another. It’s so violent that we really don’t even have atoms anymore. We just have ions and electrons bouncing around. And, you know, the very highest temperature stuff we can do that you cannot achieve by combustion, we do, electrically. I think that’s a really important point that needs to be made. It doesn’t mean, though, that using plasma is going to be fun, you know, something that you’re going to do for fun or profit in all applications, because it does have some serious downsides.
I’ve been involved in projects where plasma was used for very specific reasons, because it allowed us to produce a very specific product under the right conditions, and controlling them is fun. I mean, nerd fun. So to give you an idea, there was a project that I was involved in that had a plasma, and we had to keep the plasma from getting onto the walls of the vessel. And the way that was done was with an electromagnet that acted as a lens in order to repel the plasma and force it to be narrower than it wanted to be normally. And that electromagnet was 100 kW. This was a pilot plant, okay? And all of the energy that went into that electromagnet did not go into the product that was all lost. Right?
That was heat energy that was lost to conductors and so on to make this intense magnetic field. So when you get up to these high temperatures, you start to get efficiencies, less than 100% at converting electricity into heat. In the process that you want, some of the heat goes elsewhere, like into an electromagnetic or into switchgear or into microwaves that don’t, microwave generating equipment that doesn’t do exactly what you want it to do, and some of it goes elsewhere and so on.
MB: I care less because wind turbines and solar panels to plasma is so much more efficient than so many of the other stuff. Especially because it doesn’t heat the atmosphere.
PM: Yes. I mean, you know, when you look at it from an overall effectiveness perspective, it’s a no-brainer. And the thing that I think a lot of people have, the box that’s still stuck on their head about this issue or around this issue, is the notion that they seem to think that the piece of equipment, even if it’s a big multi-billion dollar plant, can’t change or it can’t change very much, because that would cost a lot of money. And what they don’t understand is what we engineers do is we optimize between capital costs, the costs of pieces of equipment, and how they’re arranged in space and how they’re connected and controlled and all that sort of thing. And operating cost, how much it costs to run them.
And if I say to you, well, I can keep the equipment the same, but the operating cost is going to increase by a factor of ten. Deal with it. You’re going to fire me. And you should fire me because I’m a moron for giving you that advice. No, what we’re going to do is we’re going to change the capital plant so that the operating cost doesn’t increase in this dramatic way and it will pay back in a reasonable period of time. That will make people whose money is being invested feel good about it, and that’s what’s going to happen. And those plants that can’t be modified because they’re not economic for some reason or another, they’re just going to be shut down. This is the way capitalism has always worked.
The problem that we have, fundamental base level problem that we have, is that while we permit the atmosphere to continue to be used as a sewer for all of the effluent from fossil burning, we have this illusion that we can do things with fire more cheaply than we can with electricity. When we remove that illusion from the market with carbon taxes or emission bans or a combination, then all of a sudden engineers will go, aha, I’m not going to use fire anymore. Fire’s dumb. I’m going to use electricity and they’ll figure out how to do it in a heartbeat.
MB: So I was looking at a study yesterday because I’m involved in editing the second edition of a book on supergrid technologies and stuff. The Re Institute out of Switzerland has done a more up to date stuff rather than the kind of crappy Nordhaus economic modeling around climate impacts. And it’s ten to 15% of GDP in 2050 with two or 2.5 degrees of warming. That’s the degree of economic impact.
PM: Yeah, there’s no question it’s mindblowing how expensive not having a stable climate can be on the Earth. So, I mean, and not just in money, in lives and in human misery.
MB: So this is going to get priced. We’re getting there. I spend a lot of time looking at carbon pricing schemes globally and, you know, stuff like that. But let’s get back to industrial heat. So, electric arc furnaces, what temperature ranges do they run at and what are they used for?
PM: Well, the most popular use of an electric arc furnace is for steelmaking. Actually, the majority of steelmaking in North America uses the electric arc furnaces as opposed to blast furnaces, the method that’s used primarily in places like China and India. So what we do is we start with scrap steel, and we add iron that’s been reduced by other methods, which, by the way, can be decarbonized too. That’s fed to the furnace. And then we basically stick big electrodes that are made out of graphite into that material and pass an enormous current through it. And the result is the temperatures that are necessary to melt iron. I mean, 1700 degrees C. And it’s routinely done. It’s done every day.
And the reason it’s done is that it makes perfect sense when you’re running mills that are not directly connected to a blast furnace because you don’t have giant quantities of raw iron ore coming in. But instead, the majority of your feedstock is scrap steel. And it’s a more economical way to make steel. And it makes cleaner steel and steel with tighter tolerances and all that good stuff that we want. And it doesn’t involve fire. It involves electricity. So that’s the thing that a lot of people have in their head that’s kind of backward. They talk about the decarbonization of steel making. Well, steel making is pretty decarbonized in a lot of the developed world already.
It’s iron-making. It’s reducing iron ore to iron metal. That’s the part that we do mostly still with fossils. And we do it one of two ways. We do it in blast furnaces with coke that’s made from coal. And we also do it in this process that’s called the direct reduction of iron, or DRI, where we use a synthesis gas that’s made from natural gas. It’s a mixture of carbon monoxide and hydrogen. The cool thing is you can do DRI using pure hydrogen, but you have to add electric heating.
MB: You can also do DRI with biomethane.
PM: Correct. You can do DRI just the way that we do it now, except not use fossil methane. We can use biomethane. And we can do blast furnace production using biochar as well. I mean, that’s how we made most of the iron on earth for a long time, we did not use coal. We used charcoal. And, you know, we can switch back to doing that again, too. So anyway, a lot of these high temperature things, if you think about them properly without the box on your head of fossil burning, there are ways to do it.
MB: One thing I always love is, I’m reading through a study about industrial heat or decarbonization, and somewhere it says electric arc furnaces. And then later on it says, but of course, for high temperature heat, we need to burn stuff. It’s in the same document.
PM: Kind of, you know, not to go off-topic, but another thing that comes up in this is this whole notion that we need to burn stuff, not just because we need heat, and they have it in their head that the only way you get heat is by burning stuff, but we also need to burn stuff, because otherwise, how are we going to get all these materials that we make out of petroleum and so on? And, you know, I’ve written an article, a long, painful article, rather rude article that you can find on my LinkedIn profile, which talks about what we’re really going to do in a decarbonized future to make materials and plastics and the like, and we’re going to make them out of petroleum. [The Refinery of the Future – a thought experiment] We’re just not going to do it by burning anything. It’s the same thing.
It’s electric heating and some smart uses of chemistry and the right catalysts and so on. And it’s kind of a no-brainer, honestly. It’s really a matter of how much do we want to pay and the relative cost of burying CO2 in the deep subsurface, which we know to be expensive and unlikely to get a whole lot cheaper in the future, versus making chemicals using electricity, which is another process that’s fundamentally fraught with thermodynamic difficulties. So we’re kind of between a rock and a hard place on part of the process, but it’s only part of it, and it’s manageable. It’s really not a problem. So don’t worry. You’re not going to run out of chemicals and plastics in the decarbonized future. We’ll have plenty of them.
MB: So let’s talk about aluminum. What are the temperatures for aluminum? And what’s the process for aluminum?
PM: Yeah, aluminum is not all that hot. It’s about 900 degrees C to 950 degrees C. And it’s done in a melt electrolysis. So most of the energy that goes in is not heat. Most of the energy that goes in is electricity directly, which is the process by which the aluminum oxide is reduced to aluminum, metal, and oxygen. The one thing that we have to do with aluminum that’s a little tricky is that in the past, electricity was expensive and fossil fuels were cheap. So what we did is we used, on purpose, carbon electrodes inside the melt electrolysis furnace, and we deliberately burned them. So instead of producing oxygen at one electrode and aluminum at the other, we produced carbon monoxide at one electrode or carbon dioxide at that electrode and aluminum at the other.
And by so doing, we reduced the amount of electricity that we consumed by about 30%, which was worth a lot of money. And these electrodes were, by the way, made out of the garbage from petroleum refineries. They’re made out of a mixture of residuum and petroleum coke, both of which are junk. Right. And so these plants have these huge facilities where they make these anodes out of pitch and coke, which come in on rail cars and so on. So we already know how to replace these electrodes with a ceramic electrode that conducts electricity, that eliminates this carbon dioxide / carbon monoxide business, and eliminates all of the fluorocarbons that get generated by virtue of the fact that this process is happening in return for a little bit more electricity.
There’s a company called ELYSIS, which is a joint venture of Alcoa, and another company. They have an operation that they’re setting up in Quebec, where there’s hydroelectricity in plenty and lots of aluminum plants to test this on. And they’re testing the decarbonization of aluminum. The thing about aluminum, though, is that there’s a step first that you have to do, which is you have to make alumina, the feedstock for the melt electrolysis from bauxite, which is the ore, and that process has some heating steps, which, again, are within the realm of possibility to do electrically. So decarbonizing alumina production from bauxite, I don’t see that as a major problem. That’s well within the reach of electrification.
MB: We only have ten or so minutes left. There are probably five different heating technologies we didn’t talk about so far. You started to talk about induction. So I’d like you to do a minute each or two minutes each — each of the technologies we haven’t talked about and what are their applications and roughly their temperature ranges? And these are all electric, right?
PM: Let’s start with induction. Induction only works on things that conduct electricity. It’s a way to deliver high amounts of power to metallic materials and other things that conduct electricity, like graphite, for instance, temperature range can be very high. I mean, you can heat things, metals, to white heat very quickly with an induction coil, but their efficiency is not very high. And the efficiency drops as the temperature goes up because you have to keep the electrode that actually does the, or the coil that does the induction heating. You have to keep that cool. And so you reject some heat to that cooling water. And you also need switchgear in order to produce the high frequencies that are required in order to produce the induction. But it is, it’s widely used in industry.
It’s used, for instance, to heat up chunks of steel to high temperatures before they’re forged, you know, before they’re beaten into shape. So that’s induction. Another one is microwaves.
MB: Lots of people now have induction cook stoves.
PM: You’re absolutely right. Yeah. And there you have a conductive thing, a conductive plate, and in fact, it has to be magnetic because of the frequencies that are used. And you can basically do everything with an induction cooktop that you can do with gas fire without putting nitrogen oxides into your home and giving your children asthma. That’s pretty wonderful.
MB: Here’s my favorite example recently. You know those Chinese kitchens with the massive woks and the huge jets of gas? Now they’ve got induction versions of those.
PM: That’s incredible. If you can do wok tossing cookery with induction, basically cooking is taken care of. So it’s done.
Next, microwaves. So microwaves work by exciting water molecules and some other atoms and groups of atoms in molecules. You can do all kinds of very interesting things with microwaves, including some really fascinating chemistry that happens as a result of you heating up the molecules themselves, as opposed to heating up things that transfer heat, you know, like metal things that transfer heat to the molecules themselves. So microwaves, for instance, can be used to make things like carbon black and acetylene from methane. And that’s pretty cool because those molecules have uses of a non-combustion nature in a decarbonized future. So that’s pretty interesting.
MB: And we have way too much biomethane, which we can use for that purpose.
PM: Yeah, there are limitations. And, you know, they’re not going to be a, these processes are not going to be a major source of hydrogen in the decarbonized future, but they will be a source, a potential source of decarbonized production of certain things. And microwaves are one method and plasmas are another that are used to do those kinds of pyrolytic reactions. But microwaves are, you know, they’re also largely used in things like cooking and mineral processing and various other things.
MB: A pyrolysis expert recently told me it should be called thermolysis.
PM: Yeah, well, I mean, pyrolysis. Pyrolysis. Thermolysis that, you know, really, the terminology there has to do with how you interpret the Greek. The Greek words that you start with. Right? So pyrolysis means breaking things with fire, and thermolysis means breaking things with temperature, if you will, or with heat. And so, yes, perhaps it would be more correctly called in the future, thermolysis rather than pyrolysis. But that’s getting pretty, pretty geeky, I’m gonna say.
MB: But this is a pretty geeky conversation, so…
PM: Well, in a sense, you could ultimately, if you wanted to go one step back, you could call it electrolysis. You could call it electrolysis, even though it’s not really electrolysis. Okay, so let’s keep going. Think of electrolysis. Ions moving around. Anyway, so induction microwaves, infrared, microwaves. We talked about plasma. Well, infrared is just basically transferring heat by shining light, infrared light on things, from hot things. It’s really not an electric heating technology. It’s kind of an application of heat transfer, if you will. We do use it in a lot of applications where we need to, for instance, cure inks or polymers or the like. And it’s not limited, by the way, to infrared.
I mean, we can shine ultraviolet light on things, and we can shine other wavelengths, and, in fact, we can irradiate things with other wavelengths of electromagnetic radiation to do the things that we want to do. It’s not all about heat. Sometimes it’s about exciting molecules directly, making them jiggle, vibrate, rotate, or do whatever they’re going to do in their own way. In a sense, it’s kind of just an extension of microwaves, if you will.
MB: Right. Man, I’m running out of additional ones. We’ve covered plasma, we’ve covered arcs, we’ve covered induction, we’ve covered microwaves. That covers EMF.
PM: There’s direct ohmic heating. So another example. Yeah, and the last one would be heating by doing work on something. So direct ohmic heating, as an example, would be passing a current through, as an example, a liquid metal. So if you can find a way or through a pipe, and just using the pipe to heat up the thing. If the pipes are made out of metal, you can just literally put an electrode on either end of a chunk of pipe and use the pipe as the resistor. So it’s really another way to do resistance heating. And ohmic heating is used in a lot of applications where you want to, for instance, melt something out of a pipe. You know, that’s one example.
And as far as doing work on something in order to heat it, the example that I gave at the very beginning of our conversation was around heating up plastics. So when you have something that’s really thick and viscous, sometimes getting heat into it by heating something, you know, heating a pipe, it’s so difficult to move the material through the pipe that the heat transfer that results is very poor. You know, the outside of the thing gets hot, and then thermal conductivity isn’t good enough to get the inside of the thing hot enough. So what we do instead is we basically use a mixer, and we mix the thing. We put electrical energy into a motor and use the motor to move a mixer around, whether it be an extruder or an auger or the like.
And by friction of the material against itself and against the walls of its container, we heat the thing up. And when you’re using electricity, there’s really nothing to say to not recommend that. It’s just as efficient to heat up a polymer using work as it is to heat it up with a resistance heater. There’s no difference at all. And one of them is much more effective than the other. So guess which one we’re going to use. So again, it’s all different ways to do the same job.
MB: My favorite example of that is from my buddy Swarandeep Singh, who’s an engineer with ABB, is working on the electrification of BASF’s massive new plant near Shanghai recently. So I was talking to him when he was there. He introduced me to this thing, which is basically just a drum with turbine blades in it that are turned at high speed.
PM: Right. This is tribomechanical heating. Yeah. So that’s another. I was reading about that today, and honestly, I’m still a little bit mystified about how it can work at very high temperatures because the blades themselves, you know, even though we’ve developed blades that are capable of, in jet engines and gas turbines and so on, that are capable of withstanding very high temperatures, when you’re actually using the process to do heating, it’s at a certain point you’re up into these superalloys, and even they’re not very strong anymore. What we do in engines is we actually pass cool gas through the turbine blades in order to keep the blades cooler than the thing that we’re, than the gas that’s being processed. It’s kind of limited what you can do when you’re doing that process in order to heat something up.
So, yeah, it’s an interesting concept, and one that is certainly worthy of further investigation. There’s all sorts of clever things you can do, and if you need a hot gas, you certainly do not need to produce it by means of fire.
MB: In this case, it was an olefin manufacturing. In olefin manufacturing facilities, the specific approach this persists in the temperature range longer to maximize the generation of olefins.
PM: Well, the really important thing in making olefins is that you want to hit the temperature that you want to hit, and then you want to very rapidly quench that mixture that you made in that hot place. So, because what you’re trying to do is you’re kind of trying to freeze a mixture that is away from its equilibrium. If you let it cool down slowly, you get wrong products, you get products that you don’t desire, back reactions happen, and the thing you put all this energy into making just falls apart again. So, yeah, this combination of very precise, controlled heating without hot surfaces that cause coking and charring and other things, followed by extremely rapid cooling, and you can really get a wonderful yield out of a process like making olefins.
So, yeah, lots of things that we can do better with heat, with electric heating, than we could ever do with fire.
MB: The last topic you mentioned is, we do a lot of stuff with heat from burning fossil fuels because it’s cheap. And the first paradigm is, well, what are we going to burn that’s clean? The first paradigm shift is, where are we going to get the energy we need for heat, opening it up to not burning things. The second paradigm, though, is, do we need to actually use heat at all to achieve the same outcome? So you mentioned that because if we start thinking differently, there’s different ways to achieve the same outcome. So give me your top three examples where it’s not something where we use heat today, where tomorrow, you just don’t think we’re going to use heat at all.
PM: Well, I mean, one perfect example is hydrogen production. In the present 99% of hydrogen is produced by heating up steam and reacting it with a fossil material in order to produce a syngas mixture that you then react further through catalysts in order to make hydrogen and CO2. In a decarbonized future, we’re not going to do that. We’re going to be electrolyzing water or steam and producing hydrogen that way. So that’s one perfect example. So it’s not really heat at play, it’s electricity. Another example is magnesium production. So magnesium is an extremely important metal. It’s made in a similar process, or it can be made in a similar process to aluminum, and it’s extraordinarily abundant, especially in seawater. So you can make this metal without mining, which is incredible.
The thing is, though, that you can also make magnesium metal if labor is cheap and the atmosphere is a free sewer. You can make magnesium metal by a thermochemical process called the pigeon process. And that’s actually how substantially all the magnesium metal is made in the world right now in China. And we’re aiming to fix that by switching back to electrochemical methods, greening up this metal, and providing a super additive benefit by light-weighting things that need to be light in order to be energy efficient. That’s another example. But I’m sure if we talked about it for a long time, we could think of lots of them.
MB: The third one that occurs to me is Boston Metals.
PM: Oh, yeah. So, I mean, Boston Metals is a similar thought process to what’s going on, what we’re doing with magnesium, except they’re trying to do the same thing with iron and other ores to get other metals like vanadium and the like. And, you know, the thing about doing it, doing molten oxide electrolysis for iron, is that the temperatures are so incredibly high. What Boston Metals has done is absolutely incredible. But by their own admission, they’re at least a decade away from being commercial. So, you know, I’d wait until we actually had some chicken eggs before we think we’re going to have chickens. In that regard, I think we’re a little premature to conclude that’s going to work out okay. But anyway, we can be hopeful.
PM: There are also electrochemical methods at room temperature or at low temperatures that can be used to produce iron and other metals. So, yeah, switching electricity for heat or for chemical reactions that happen at high temperatures is definitely de rigueur. It’s something we’re going to do in the future.
MB: I’ve seen, as I think I said recently, I’ve gotten to the point where I’m no longer completely incompetent in chemistry. But I know a lot more about what I don’t know. And for electrochemistry, I’m nowhere near that.
PM: Electrochemistry is really interesting, man. One of the things that blew my mind was just how important a small fraction of a volt can be, and what the equivalent is in thermal terms to a fraction of a volt. I mean, the difference between 3 volts and 4 volts DC is a difference of hundreds and hundreds of degrees Celsius in terms of thermal reactions. So it’s really interesting that whole different classes of reactions happen at 4 volts that don’t happen at 3 volts, and reactions happen at 3 volts that don’t happen at 2 volts, and so on.
MB: Yeah, it’s fascinating. I won’t get into specifics, but I see a lot of value propositions where we’re going to start using electrochemical processes instead of processes with a lot of heat in the future, and it’ll just be a lot more efficient. But we have to think differently about the problem. So, first paradigm, get rid of the burner box, as you say. Second paradigm, say, do we, can we get to end without heat at all? And so, okay, Paul, you know the drill. You’ve done this a couple times before. This is your open-ended opportunity. You’ve already pitched Maghrea, or magrathatha, or magretza, or whatever the heck it’s called.
PM: Magrathea. We are the people that make planets. We’re planet-makers.
MB: So you’ve already pitched them. So I’m going to give you an open-ended opportunity for anything else you want to tell people.
PM: I’ll just say that there are a lot of people out there who are motivated to tell you that electric heating is hard. I’m here to tell you from practical experience for decades and decades, it’s not hard, it’s just different. And the thing that’s hard is the value proposition. And we as a society have to decide to create the value proposition. Once we’ve done so, people like me will go off and make it happen. It’s not hard technically. It’s not difficult economically.
MB: Excellent. Thank you, Paul. My guest today has been Paul Martin, founder and chief guy at Spitfire Research. Reach out to him for all your deeply nerdy chemistry process engineering needs and consultation. If you’re a venture capitalist or an investment fund who needs to know if something really makes sense, Paul’s one of the key guys to go to. Take care all. Until next time.
Have a tip for CleanTechnica? Want to advertise? Want to suggest a guest for our CleanTech Talk podcast? Contact us here.
Latest CleanTechnica.TV Videos
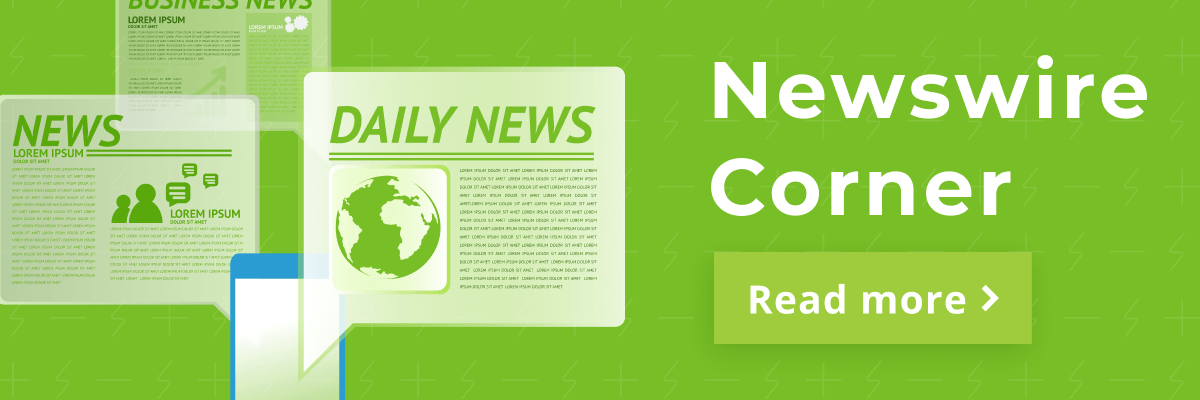
CleanTechnica uses affiliate links. See our policy here.