Decarbonizing Industrial Heat Means Electrifying It — Transcript of Podcast With Paul Martin
Recently I sat down virtually with professional chemical process engineer Paul Martin of Spitfire Research on my podcast Redefining Energy – Tech to discuss how much industrial heat can be replaced with electrical heat technologies (tl’dr: virtually all of it directly). In the theme of providing transcripts of presentations I’m giving in various places for people who prefer the written word, this is the lightly edited transcript of the first half of our conversation.
Michael Barnard (MB): Hi, welcome back to Redefining Energy – Tech. I’m your host, Michael Barnard, and today I have Paul Martin back. Father Paul, as a client was just sharing with me, and today we’re not going to spend an hour and a half slamming hydrogen for energy. It may come up. We’re going to talk about industrial heat and specifically electrification of industrial heat in all its glory. I’ll give the brief summary on Paul and then he can explain more. Paul’s been using electric heat for industrial processes professionally for decades, building big modular chemical engineering plants. He uses electricity until he doesn’t have to because of business cases. So, Paul, welcome back. And as always, tell the people who just don’t know you yet why they should know you.
Paul Martin (PM): Well, thanks for the introduction. I am a chemical engineer with about three decades of experience working largely in the chemical process development space. So designing for 26 years, designing and building pilot and demonstration scale plants for the world’s new chemical process technologies in energy and chemicals, in materials, in primary metals and like. And what’s my qualification for today’s discussion? Well, I have designed and built and been involved in the design and construction of a great many process plants for every kind of process that you can think of.
In that history and those dozens and dozens of plants that I’ve been involved in, we used fire to make heat in exactly one of them, and all the others provided their heat by means of electricity, or in one other case by means of electricity indirectly by virtue of doing work on the thing that were trying to heat up. So basically running a polymer material through an extruder that was designed to heat it up as well as move it. So that’s my experience. A long list of projects where we used electricity for heat because at the pilot and demonstration scale, fire is dangerous. It’s complicated and it doesn’t save enough money by using a cheaper fuel versus more expensive electricity to merit the capital cost of using fire.
MB: So we don’t use fire, but that doesn’t mean the plants, when they’re built in scale, don’t use fire.
PM: That’s correct. The reason we use fire in industry is that if we’re going to make electricity using fire, it would be crazy to use that electricity to make heat. Although we do that in certain instances, certain applications, electric heating is still used where it makes sense, despite the fact that we’re going through this kind of ridiculous path of taking chemical energy from petroleum or natural gas, converting it into heat, and then using that to make electricity, and then transmitting the electricity, and then using electricity to make heat again in a resistance heater. It seems nuts, but we do that when it provides an effectiveness benefit. But of course, in a decarbonized future, we’re not going to be burning chemical energy to make heat, and we’re going to be starting with electricity.
And it will be just as dumb to take electricity and use it to make a fuel, which you then use to make heat, instead of just using the electricity to make heat, either directly or by heat pumping.
MB: So let’s start with exergy 101. You know, talk about exergy and this subject, just because most people don’t get this yet.
PM: Sure, this is where Father Paul gets to preach a little bit about committing sins against the laws of thermodynamics. Exergy is kind of a complicated topic, and it’s not taught very well, even in university, but it’s really simple to understand. Basically, exergy is the potential of a unit of energy to do work, to provide mechanical energy, for instance, to move something around. And the exergy potential of a unit of energy varies depending on what it is. So the exergy potential of a unit of electricity is really high because we can convert electricity with high efficiency into whatever we want, whatever other form of energy we want, with reasonably high efficiency. The exergy potential of a unit of heat depends on how hot it is and how cold the surroundings are.
It depends on the difference in temperature between the hot place and the cold place. If I have a unit of heat energy at room temperature, and I don’t need to keep the room warm, in fact, I need to pump heat out of the room to keep it comfortable, that has no exergy associated with it. It’s in fact, worse than worthless. The analogy I like to use with respect to types of energy and their exergy potential is comparing them to currencies. If I say that I have $100, that’s great, but it’s not sufficient information. I haven’t told you whether I have 100 Jamaican dollars or CAD 100 or 100 American dollars. And the exergy potential of a unit of energy is just like that.
Just because something is a unit of energy measured in joules, doesn’t mean it’s worth the same. Just like if I have a dollar and I don’t tell you what kind of dollar I have. I don’t necessarily convey to you how much value I have in my hand or my pocket. The really cool thing about exergy is, if you have pure exergy, like electricity, you can use it to do work. The really cool thing about that fact is, not only can you do useful work, like moving things around, you can also use it to move heat around in the opposite direction to the way it normally flows. You can pump heat out of your beer and use it to cook your eggs, if you so desire. Now, you can’t do that for free.
You can’t just switch the direction that heat flows spontaneously. You have to do work in order to pump the heat out of your eggs and use it to cook something else or out of your beer and use it to cook your eggs. That doesn’t happen spontaneously. Anybody who says that it does is committing a sin against the second law of thermodynamics. And thou shalt not sin against the second law. The second law is important.
MB: One more thing to say, just to make it really clear. Electricity has high energy, but it also, if you need heat, it also turns directly into this one unit of heat.
PM: Yeah, thermodynamics does not prevent you from converting pure exergy to pure heat with 100% efficiency. Now, in practice, if you want really high temperature heat, thousands of degrees, the practicality of making that heat with devices that survive under those conditions do sometimes involve some losses, like electrical switch gear losses, or making electromagnetic radiation of some other kind that doesn’t end up as heat in your device or whatever. But, you know, these losses tend to be fairly small. So, yeah, the worst you can do with a unit of exergy is turn it into a unit of heat at any temperature you want, which is the thing that I think people miss.
MB: Let’s start talking about temperatures of heat and chemical processes. Let’s talk just about stuff below 200 degrees. I’m not a professional industrial engineer or chemical process engineer. I just respect it and understand it well enough that you don’t hate me when I say stuff about it. I think that’s the best way to describe Paul’s opinion about my STEM competence. He appreciates the fact that I try.
PM: Well, you try harder than most people, Michael. You do more with less preparation than most people do, I can tell you that. The thing about the temperature.
MB: Yeah, under 200 degrees, how do you separate that out? How do you separate out under 200 degrees as a professional?
PM: Well, there’s actually two pieces to this. This, by the way, is all of comfort heating, the heating that we use in order to keep ourselves warm in cold climates. And a large fraction which varies from place to place. But somewhere on the order of about 40% of the joules of industrial heating that are used at temperatures below 200 degrees C. And why is 200 degrees C a magic number? Well, it kind of isn’t. As I mentioned, there’s two pieces to this piece. Number one is the upper temperature, and that has to do with practicalities of moving heat around with heat pumps, which we’ll talk about in a minute. But the other one that’s actually more important is what we would refer to in a heat pumping situation as temperature lift.
Obviously, heat naturally flows from hot things to cold things, because the molecules in the hot thing cause the molecules in the cold thing to jiggle around faster, and heat is transferred that way, if you will. A perfect example would be, let’s say that I have a distillation that I’m running. I want to boil something, cause it to form a vapor, and then contact that vapor with liquid that I condense at the top of a distillation column, and by the interaction of the vapor with the liquid, cause things that boil at lower temperatures to leave with the condensate from the top of the column and things with higher boiling points to stay in the bottom of the column.
In that instance, almost every joule that I put into the boiler at the bottom of that distillation column comes back out the condenser again just at a lower temperature. Now, of course, we can’t cause the heat that we’re rejecting to cooling water, generally in the condenser, to jump up in temperature of its own accord to be hot enough to heat up the boiler. But we can use work to pump heat from the condenser back into the boiler. And we can do that one of two ways. One way is by using a refrigerant and compressing it and then cooling that refrigerant and causing it to condense. That’s basically the process of refrigeration that we use in refrigerators and air conditioners and things like that.
That kind of a process tends to have an upper temperature limit of about 200 degrees C on the hot side, because the hot stuff that comes out has to come out hotter than that from a compressor. Compressors are made out of materials of construction, and at higher temperatures, those materials of construction start to become troublesome. And also the working fluid, the thing that we’re using, the refrigerant that we’re using to do this compression and condensation and evaporation cycle, tends to, there are more choices at lower pressures and so on at lower temperatures than there are at higher temperatures. But the other way that we can do this is we can use the process fluid as the refrigerant.
So, in the case of the distillation column, what we can do is, at the top of the column, instead of condensing the vapor, we can feed the vapor into a compressor, and the compressor will then increase the pressure of that vapor and will not cause it to condense. In fact, it will always superheat that vapor. Even though the pressure is going up, it will put some energy into that vapor that will result in the vapor being superheated, meaning it won’t be ready to condense until it cools a bit. We can then take that hot vapor at higher pressure and put it into the boiler of our distillation column and use it to cause boiling.
As that vapor condenses at its new higher pressure, and hence higher temperature, it will transfer heat into the liquid in the reboiler and cause that liquid to form the vapor. We’re actually using the process fluid as the refrigerant. We’re doing this compression, condensation, and then evaporation cycle in order to pump heat around. That has a special name, and it’s called mechanical vapor recompression. The nice thing about all of these schemes, whether they be using a refrigerant for conventional heat pumping, or whether they use the process fluid as the refrigerant in mechanical vapor recompression, they give us what’s referred to as a coefficient of performance.
What coefficient of performance means, in simple terms, is it’s how many joules of heat I get to move from the cold place to the hot place in return for how many joules of electrical energy that I feed in. So, if, as an example, I feed one joule of electricity to a heat pump, and in return for that one joule of work, I get three joules of heat to move from the cold place to the hot place, I have a coefficient of performance of three divided by one, which is three. And with mechanical vapor recompression, you can get, depending on how high a difference there is between the hot place and the cold place, that temperature lift, as they call it, you can get coefficients of performance that are very high, like five or more. That’s pretty cool.
You know, not very often can you, with a straight face, say that you have a process that has an efficiency, if you want to call it that, of 500%, and not be a liar or a grifter or a thief or whatever. So, yeah, anyway, low temperature processes, which make up about 40%, and in some places, more than 60% of the joules of heat that we move around, tend to be within reach of heat pumping schemes, whether they be conventional heat pumps or mechanical vapor recompression. As a result, they’re going to be very easy to electrify, meaning inexpensive to electrify. In terms of operating cost, relative. However, that’s relative to using electricity directly to make heat.
It’s not relative to burning fuels, because right now we have this giant atmosphere that we dump stuff into for free or nearly free, and we think that’s, you know, a good deal. Of course it’s not. It’s. It’s basically robbing Michael to pay Paul, for instance, if you will.
Well, actually, in this case, we’re robbing ourselves and pretending that we’re paying ourselves, because we’re basically ruining the climate for future generations by our stupidity. So at the end of the day, we have to figure out the difference between cost and value and do the right thing. When we get around to doing that, the good news is technology is going to be there to help us, in this particular instance, for that chunk of heat below 200 degrees C, and especially where heat is being rejected to a cooling medium, and we need to put heat into something else. We can couple the two, whether they’re in the same process, like distillation, or they’re in different processes, it’s going to be actually quite easy. It’s routine chemical engineering. It’s not difficult to do.
The reason we don’t do it is that burning stuff is cheap, right?
MB: Two things here. One is that under 200 degrees, that’s still steam forming, water is boiling at those temperatures. Just so anybody who’s missed that part of the conversation, this is Celsius, not Fahrenheit.
PM: We don’t speak Fahrenheit.
MB: No, while we’re talking thermodynamics, we’re not going to use the Fahrenheit scale. Thermodynamics, Kelvin. This is Celsius. That’s statement one.
PM: That’s right.
MB: Boiling water. We’re well above the point of boiling water. The second thing, though, tell us, you’ve said distillation column, which in your mind, you’ve probably built dozens of these, again, for different things. But give some examples of distillation columns, like start with the oil and gas industry.
PM: Where we really should start is making whiskey or vodka, whatever your drink happens to be. Fermentation of whatever you’re starting with gives you a mixture of water and ethanol and some other molecules, some of which are bad, give you a bad hangover, or may even make you go blind, or worse, cause cancer and other things. We want to separate that mixture, and we want to put the good stuff in a bottle and drink that, and we want the bad stuff to go away. That separation process that’s used is distillation. We boil the liquid, and the light vapors that come off first contain some nasty molecules. We condense those and throw those away, and then we start getting the good stuff, and we collect those, and we call that the hearts of the distillation.
Eventually we start getting stuff that starts to taste like cardboard or wet dog or things like that. We call those the tails, and we might throw those into the next batch. That’s a batch distillation. Most industrial processes don’t do distillations in batch. They do them continuously. They will feed a mixture to the middle of a distillation column. They’ll continuously boil stuff up at the bottom and continuously take stuff that doesn’t boil away from the bottom. At various points up the column, they will take off things of value, and they’ll do that on a continuous basis. An example of that is petroleum distillation, where we actually do, well, a number of distillations, but two primary ones. First we do, we take crude oil and we desalt it, and then we distill it at atmospheric pressure.
The stuff that doesn’t boil at atmospheric pressure, we feed to a vacuum distillation column. We actually lower the pressure so that we can lower the boiling point of various species. The very bottom of that column, of the vacuum distillation column, we call residuum, or resid. That’s the basically liquid tar, the very bottom of the barrel that we use to feed to ships. We still use it for road tar and roofing tar and things like that. There are lots and lots of distillations in chemical plants that are separating valuable products from feedstocks or products from reaction mixtures and so on. They’re used all over the place. They use a tremendous amount of heat, and most of them reject their heat to a cooling medium..
We chemical engineers are fairly smart about using this hot stream to heat up another stream and hence cool down the hot thing that we don’t want to be hot anymore. We’ll do cross exchange from place to place whenever that’s practical, and we’ll have whole heat networks set up. That’s actually where the steam business comes from. You were talking about steam a minute ago. A lot of the reason that we use steam is as a way steam becomes kind of a currency for moving heat around. It’s used to allow us, for instance, to extract heat from one process and transfer it into another indirectly, without the two streams having to come in contact with one another or even share the same space in the heat exchanger, even though they’re separated by metal from one another.
The steam acts as a kind of a transport medium for that heat. A lot of that steam use arises, not because we really need steam, but because when you burn things, you always end up with this hot flue gas that you have to get rid of. That hot flue gas, in order to avoid throwing it away, you put it through coils and use it to make steam. Or you put coils of boiling water in the flue gas in something called a heat recovery steam generator, and you use that to extract heat from that flue gas before it goes on to the atmosphere.
In a decarbonized future, a lot of the steam business that we’re using right now will go away, and all of its nuisance and capital cost and operating cost and maintenance cost and corrosion and blah, blah, blah, will go away. Honestly, in these small plants that I built for many decades, that was one of the big reasons we used electric heating, because we hated steam. Steam is a nightmare. It’s a nuisance, and if you don’t need to use it, you don’t use it.
MB: But let’s just do some basic thermodynamics education. Why does steam contain so much more heat than water?
PM: Well, I wouldn’t say that, the way I’d say it is this. The nice thing about steam is that you can transfer a lot of heat at a constant temperature. At a given pressure, water vapor condenses at a given temperature. If you start out with pure steam, you can reject heat from that steam and gradually form condensate until all of the steam is gone and the temperature won’t change. That what’s referred to as latent heat of vaporization, or of condensation, if you think of it the other way, that’s extremely valuable, because it allows a small amount of matter moving around in order to transfer a very large amount of heat.
If you want to do that with what we call sensible heat, which is, for instance, let’s say we have water that we heat up to 80 degrees C, and we let it drop back down to 40 degrees C by heating other things up. We have to move a lot of matter around in order to deal with only that sensible heat. If we, for instance, instead have atmospheric pressure, steam, or better still, you know, steam at about one 1 bar or 1 atm above atmospheric pressure, and we condense that, we’re moving an awful lot more heat with an awful lot less matter. That’s a good thing. It makes the carrying of heat from place to place more efficient.
MB: Paul’s problem is that he’s forgotten more than most people have ever learned about thermodynamics. So I’m going to step back to a really basic statement, something that escaped scientists for centuries, which is when you heat water up, it gets to 100 degrees celsius and then stops for a long time. More heat is poured into it until the phase change occurs. So, same with when you freeze it, you get it down to a certain temperature, and it just stays at zero celsius for a long time, until ice crystals start to form. And so that stuff about that latent heat of vaporization, and Paul is waiting to correct me, I know, but when he’s talking about the steam at 100 degrees, steam just sits there and emits heat for a long time as it slowly turns to water, and then the water gets cooler.
But at that phase change boundaries, the temperature persists for a long time. So now do the different, better nuanced version of that, Paul, because people don’t know that.
PM: No, I think the second way you put it was a little clearer. What doesn’t happen is we don’t put energy into water at 100 degrees C and have nothing happen and the temperature not go up. What does happen is once we’re at 100 degrees C, if we’re atmospheric pressure, as we put heat in, that heat doesn’t go into raising the temperature. It goes into boiling water and turning it into steam. The temperature doesn’t change, but the phase changes. The water molecules that used to be bouncing around next to one another in a liquid are now bouncing around far apart from one another in a vapor phase. Until every drop of water is gone, the temperature is not going to increase. The same is true on the reverse.
If you take a cloud of steam and it’s at constant pressure, it will condense at constant temperature until all of the steam is gone. And we have liquid if the pressure is held constantly. This is the great thing about steam. There are lots of other things that we can use in place of water. I mean, we use water because water happens to have an incredible heat of vaporization, and it’s also nontoxic and abundant and very cheap. But we, in the process, industry, we use other molecules to transfer heat as vaporization energy and condensation energy at higher temperatures with lower pressures, as an example. So we’ll use these molecules that Dow has formulated that they call Dow therms.
There are various Dow therms that are used as hot oils, liquids that you just heat up and cool down and use the sensible heat. There are other Dow therms that you use for their vaporization. What that allows us to do is to go up to higher temperatures without going up to very high pressures. There is some hope, by the way, this 200 degrees C thing, it could easily be 300 degrees C. That’s really a matter for innovation and changes to equipment and the use of different things, like molecules like these Dow therms as an example, instead of using things like water vapor or CO2 or the like.
We can push that envelope a little bit, but we’re not going to 400 degrees C because at 400 degrees C, organic molecules start falling apart too quickly for us to like.
MB: Let’s talk about this, because this is important. A lot of people say, well, at 200 degree heat, that stuff’s all technology readiness level six or seven. It’s not really commercialized. It’s not really here. How do you respond to people who say that, Paul?
PM: Oh, my goodness. I’d say that they just aren’t paying attention. There’s a difference between something that has a low technology readiness level because people haven’t done it before, and something that has a low technology, apparent technology readiness level, because it doesn’t have a market. There’s a huge difference between those two things. I mean, heat pumping is routine. Everybody’s got a heat pump or several of them in their house already. They’ve got a refrigerator and a freezer, and they’ve got an air conditioner, and there’s nothing magic about them. They serve a purpose, and people more or less see the box and don’t understand what’s going on inside until they have to call the repair person to come fix it. Heat pumping is, you know, the highest technology readiness level you can imagine.
Do we use heat pumping in industry? Sure, we do. Generally for cooling things, not for heating things. Why? Because burning stuff’s cheap, if you can dump stuff to the atmosphere for free. When that paradigm shifts, like a light switch, all kinds of operations where people are boiling things, cooking things, drying things, etc., will, like a light switch over from burning stuff to make that heat to using electricity to make that heat in this kind of heat pumping way. Those will happen first because they’ll be cheaper. Once we get above that number, 200 to 300 degrees C, and we’re into difficulty with heat pumping, now we’re into lower efficiency approaches. By lower efficiency, I mean not better than 100%.
MB: Natural gas burning stuff isn’t 100% either, because you have to extract it, process it, distribute it, and then there’s methane slippage, I’ve discovered in burning methane. It’s not a one for one. Electricity is actually more efficient on a one to one basis. The efficiency of electricity turning to heat is higher than the efficiency end to end of the alternative, as long as we’re not burning stuff to create the electricity in the first place.
PM: Not to bore people, but there’s an important little distinction here, and this is a problem that people run into. I find this a problem, even among engineers, that we have two kinds of heat from burning stuff, metrics that we use. One of them is called the higher heating value, and the other is called the lower heating value. Why do we have to have two? Then, of course, we have two different names. We will call the higher heating value the gross heating value and the lower heating value than net heating value. What a mess.
The thing that’s involved here is that in some cases, we can wring every little bit of heat out of the burning, out of the products of the burning, by condensing the water vapor that’s formed when we burn methane, for instance, we get CO2 and we get water vapor. If we can condense the water and produce water liquid, we get the heat of vaporization of that water back. When you take the feed materials and we let them burn, and then we cool everything down to 25 degrees C, the net amount of heat that we get is what’s called the gross or the higher heating value, because we’ve extracted every little bit, including that heat of condensation. That’s the right metric that we use when we’re looking at comfort heating.
If you say that you have a natural gas boiler or water heater like the one in my house, that has annual fuel utilization efficiency of 95%, that is relative to the higher heating value of the natural gas that’s being fed. For every joule worth of higher heating value of natural gas that’s fed to my boiler, I get 0.95 joules of heat in my house. That includes condensing and cooling down the water of combustion. But if I want to use fuel to heat up something to 200 or 300 degrees C, the heat of vaporization of the water is not accessible to me. It’s gone, it’s lost. Now what we do is instead of thinking about efficiencies in terms of the higher heating value, because in a sense, that’s kind of not fair to the device.
It’s saying, well, you didn’t catch this thing that you couldn’t possibly catch. We switch bases, you switch to the lower heating value, and that ignores the heat of vaporization of water, as if we don’t care about it. And the problem is that, you know, in energy terms, that’s real energy. There’s real energy in there, and it’s lost. We’re not using it because we can’t recover it, because we’re using the heat that we produce at too high a temperature. So one has to be very careful when looking at efficiencies to say, well, was that calculated on the higher heating value or the lower heating value? And was the one that we used at the beginning of the calculation the same as the one that we used at the end of the calculation? Or did we switch bases between those two things?
I’ve seen, people, including engineers, screw that up and they draw wrong conclusions. So you’re absolutely right. Natural gas takes energy to drill wells, to clean up the natural gas, to get it to pipeline spec, to compress it, to transmit it across long distances, to get it to somebody that needs it, dropping in pressure to the point where they start using it. Most of that compression energy is wasted, by the way, and then we burn it. Then a lot of the time we don’t capture the heat of vaporization or the heat of condensation of the product water. We’re losing tons of energy. With electricity, it’s easy. I mean, you feed it into a resistor, it makes heat with 100% efficiency, and the transmission losses are around the same as the transmission of natural gas.
They’re both about 95% efficient if you look at North America. They’re pretty similar. The notion that electricity is lossy to move around is not accurate. It’s actually quite efficient to move around if you design the distribution system and the transmission system correctly, which we tend to do.
MB: Let’s talk about a lot of people’s favorite subject, food and industrial food preparation. What are the heats that are mostly seen there, and are they all in this range? Are they mostly in this range?
PM: Most of the heat is in the cooking range, so it’s in the below 200 degrees C type range. Some of the heat in food preparation is in the frying range. There we’re just going to use electricity directly to make heat, and we can produce any frying temperature we want. When you look at industrial heating, as I said, about 40% — round numbers — is used below 200 degrees C, and that includes most of the food processing industry.
MB: People occasionally ask me, well, what am I going to do to dry my chilis in the future?
PM: I think maybe this would be an appropriate time to bring in another thing. We’ve been talking about energy. We’ve been talking about joules. We haven’t been talking about energy delivery rate, which we call power. We haven’t been talking about joules per second or watts. One of the things that’s a little bit challenging, and I say a little bit challenging, but in some cases is very challenging, is that, natural gas as an example, it’s very easy to deliver a very large heating power to an application. I happen to be working with a startup that I’m an investor in, an advisor to, and they’re doing a lot of heating because what they’re trying to do is to produce magnesium metal from seawater.
They have a laboratory, and their laboratory only has so many amps of electrical service coming in, and hence, it can only deliver a certain number of kilowatts of power to the facility. When you want to do something, like, for instance, heat up a batch of metal to its melting point very quickly so that it doesn’t hang around and have time to oxidize, you need a lot of power. You need a lot of kilowatts of energy. Delivering that through a small electrical line is challenging. Delivering it through a comparatively small natural gas line isn’t so challenging. In fact, you can have a barbecue cylinder and deliver, you know, 100% heat for a short period of time if that’s what you want to do.
Sometimes heating applications are very high power, but very low in terms of the total number of joules of energy that are delivered. That becomes a bit of a problem for electrical energy.
MB: Well, I’ll compare and contrast. A week or two ago, I was looking at, as I’m doing now professionally, the stunning drop in battery prices per kilowatt-hour.
PM: Very important topic, and one that I’m watching very carefully myself and with glee, by the way.
MB: As a battery optimist, I’m stunned.
PM: I am without question a battery optimist, just like you are.
MB: I’m stunned by the rate of change of drop. At the beginning of this year, we were under $100 per kilowatt-hour, which was kind of expected for 2030. CATL has announced LFP, lithium iron phosphate batteries, at $56 per kilowatt-hour in the fourth quarter. There are people selling them for $47 per kilowatt-hour on the market.
PM: We’re at way below wholesale prices of raw cells. People have to understand that obviously, when you buy something wholesale, you’re talking about buying large quantities of it. But you and I can buy, because I just did a couple months ago, we can buy lithium iron phosphate cells from China, including delivery, for $90 per kilowatt-hour. Because we bought some. We bought 40 cells, 280 bricks that are basically a kilowatt-hour of storage each and paid under $90 US per kilowatt-hour of battery storage that those batteries represent. That includes the rather expensive cost of shipping them from China to Canada door to door, including whatever tariffs and taxes are charged along the way. Those batteries, I mean, it’s incredible what their performance, what they will do, what they’re guaranteed to do.
The batteries that we bought have a guaranteed cycle life of 6000 cycles. And when you do the math on that, up at the farm where I’m using my portion of that battery order, those batteries are returning every kilowatt-hour that I feed to them for about two and a half US cents per kilowatt-hour plus whatever I want to pay myself interest on my investment to buy them. That’s ridiculous. How much cheaper does storage have to get than that? And by the way, it’s getting cheaper. A lot cheaper. I did the math. I love this, it’s wonderful.
MB: I know, I’m stunned, but I did the math for heavy road transportation. One of the subjects I’ve spent a lot of time on in the past couple of years, and I just said, okay, well it can take five years to get a 5-megawatt connection for a truck stop because that’s infrastructural change. But at these price points, I can put a honking big battery on there and it’ll pay itself off in 17 months just with price arbitrage and nothing else. Never mind the retail cost, sale of electricity versus the other stuff and that for heating.
PM: I’ve got a good friend who took the majority of that battery order that we just made. He has a solar installation on his house which gives him really wonderful power in spring and fall, but in the summer there’s too much tree cover that shades his roof and in the winter he gets snow on his panels and it’s a bit annoying to get it back off again. And of course the solar intensity is quite low. But what he finds his battery most useful for is that because Ontario, where I live, built this huge fleet of nuclear power plants and also has all this run-of-river hydropower where there’s no dam, where you can store energy, there’s just a weir.
And the water, you know, if there’s excess water, it flows over it because we have these resources that you kind of can’t turn off or can’t afford to turn off. We are given electricity, and I mean practically given electricity at very cheap rates at night. I’m using it to charge my electric car and it’s fantastic. It dropped my cost of driving to 70 cents per 100 kilometers, in terms of energy relative to my Prius, which was costing me $7.50 per hundred kilometers for energy. So that’s great. But what my friend was interested in doing was storing some of that energy at night and using it during the day to run the rather large hard disk array that he has to keep spinning all the time, which was costing him a bit of money.
What he did is he bought a fairly big battery and he has a system that looks at day ahead, forecasting for whether it’s going to be a sunny day or not, and does a number of calculations and then says, should I charge up the battery tonight on cheap electricity from the grid or should I not? And wait for solar to come in and fill the battery during the day, and then I’ll bleed that off at night. He just has a little home assistant with some code that in fact, I think he got AI to mostly write for him.
MB: ChatGPT writes much better code than I ever did.
PM: It does the calculations and it looks at it and says, okay, well, here are all the factors, here’s the state of charge of the battery, here are the electrical prices. It does a little bit of math. He came up with an algorithm. The code was implemented to just control all the devices. Control is inverted. Control this charge controller, and let’s move back from that.
MB: Well, it’s fascinatingly nerdy. It doesn’t talk about heat. The big point here is that for some of these points where you need a lot of power in a short period of time, we can now put batteries in at a price point where it starts to make sense for some applications. But it doesn’t help as much when you need 5 MW of heat 24/7 or 20 MW of heat, because you can’t store it.
PM: That’s right.
In the second half of our discussion, Paul and I dive into storing heat for industrial processes and several other key topics.
Have a tip for CleanTechnica? Want to advertise? Want to suggest a guest for our CleanTech Talk podcast? Contact us here.
Latest CleanTechnica.TV Videos
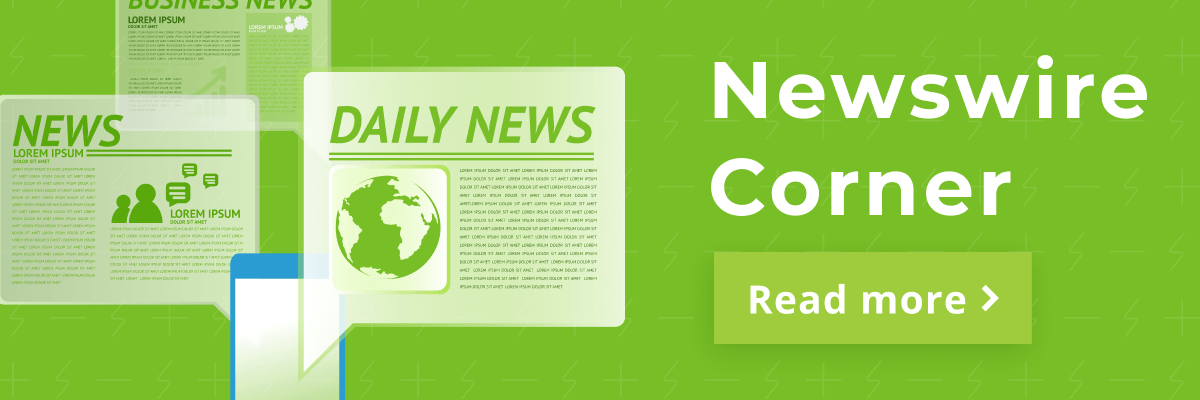
CleanTechnica uses affiliate links. See our policy here.